When we think something is 100%, we typically think it is the best it can be, that is perfect. One way of rating an engine’s breathing ability is called Volumetric Efficiency. Typical volumetric efficiencies at full power (wide open throttle or WOT) are in the range of 70% to 85%. At part throttle conditions, like cruising down the road with the throttle plate nearly closed, the volumetric efficiency can be as low as 20%.
However, a full race engine naturally asperated (no turbo or supercharger) can easily get volumetric efficiency levels over 100%, even over 130% in “perfect” conditions. How is this possible?
It’s easy to see why volumetric efficiency would be less than 100%. The engine is hotter than the incoming air, which reduces it’s density, and the amount that can fit in the cylinder. Any air restrictions going into the engine or exhaust restrictions going out of the engine further reduce air flow. Then you have the typical intake closing event at seat timing (when the valve actually seats the valve and completely seals) which can easily be 60 to 100 degrees after bottom dead center (BDC). That means the intake valve doesn’t close until the piston is maybe 30% back up the bore, meaning 70% volumetric efficiency should be the best possible (100% – 30% = 70%).
With all this working against volumetric efficiency, it’s amazing we can do much better than the 70-85% mentioned earlier. And, if engines ran at about 100 RPM, that would be the case. However, at high RPM there are all sorts of dynamics going on that really help the engine breathe. These dynamics involve the air in the intake port and runner, and exhaust port and runner (header primary), which produce tuning pulses.
In simple terms, once the “slug” of air in the intake runner starts moving toward the cylinder on the suction stroke, it wants to keep moving toward the cylinder even past BDC. The same is true on the exhaust side. The exhaust starts moving away from the cylinder down the primary, it wants to keep moving away from the cylinder. This is called inertia tuning.
I’ve included some graphs from our Engine Analyzer Pro to illustrate these points. These tuning pressure graphs can be produced as the Engine Analyzer Pro calculates the simulated engine’s volumetric efficiency at the various RPMs. The horizontal axis (X axis) is crank degrees. Important points in the 720 degree cycle are marked off, like TDC (top dead center), BDC, EO (exhaust opening event on the cam), IC (intake closing event on the cam), etc. To the right is a simple piston and valves diagram so you can visualize where in the 4 stroke cycle you are at when these graphs are being drawn.
The top graph shows intake (turquoise) and exhaust (dark red) port pressure, and cylinder pressure (gray). The blue dotted line is atmospheric pressure. Any pressures above the line are positive, high pressures. Pressures below the line are actually a suction pulse.
The bottom graph shows flow by the valves. Flows in the correct direction (which improve power) are shown above the 0 pressure line. This intake flow (turquoise) which flows into the cylinder and exhaust flow (dark red) which flows out of the cylinder. Reverse flow (which hurts power) happens when the graphs fall below the zero velocity line.
The graphs are shown in pairs, one with normal length runners, of about 12 inches on the intake (port and intake manifold) and 34 inches on the exhaust (port plus header primary). These graphs show the normal pressure pulses found in a race engine. The comparison graphs are for very short runners, 2 inches on the intake and 4 inches on the exhaust. The Engine Analyzer Pro’s software will not run with zero length runners. However, these runners are short enough to illustrate approximately what would happen with zero length runners, which would eliminate all the tuning pulses we’ve been talking about.
The graphs below show that when the exhaust valve first opens, there is a huge outflow of exhaust, called the “blowdown” pulse. After that, the piston acutally pushes the rest of the exhaust out. Both of these actions gets the exhaust flowing out of the cylinder, down the exhaust header primary.
(Click the images to enlarge them.)
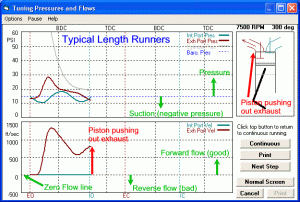
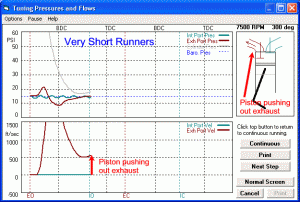
The next set of graphs (below) show that at TDC overlap (both intake and exhaust valve are open) that the Typical 34″ exhaust header keeps the flow moving out of the cylinder. There is also a suction pulse set up in the exhaust port. This is created by the inertia of the exhaust flow wanting to keep flowing in the exhaust direction. However the very short exhaust primary shows that there is no suction pulse, and the exhaust actually flows back into the cylinder (reverse flow). There was not enough exhaust flow inertia to keep the exhaust flowing in the out direction.
On the intake side, the piston is at its approximate max velocity sucking in air and fuel. With the Typical 12″ runner, you can see a suction pulse being created in the intake port. With the very short 2″ runner, the suction pulse is much smaller. Both runners show an intake flow being high and in the correct direction.
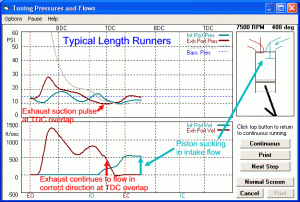
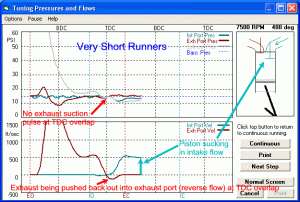
The set of graphs below now show the pressures at the most critical point in the 4 stroke cycle, intake closing. With both length runners, the piston got the intake air moving toward the cylinder. However, with the very short runner, there was not enough inertia to keep the air flowing into the cylinder once the piston stopped and changed direction at BDC (bottom dead center). After BDC the piston actually pushed the intake air back out into the intake port (reverse flow). This condition produced about 90% volumetric efficiency. If we truly had zero length runners, to eliminate all inertia tuning, the volumetric efficiency would be even much lower than this.
With the Typical 12″ runner, there was enough inertia to keep air and fuel flowing into the cylinder. There is no reverse flow, and you can see a 7 psi pressure pulse occuring during this time. (Baro press is about 15 psi, and this green pulse is well over the 20 psi line.) That is almost the same as having a supercharger installed which can create 7 psi of boost. This engine produced 111% volumetric efficiency, more than a 20% increase in volumetric efficiency, just by having typical length ports and runners.
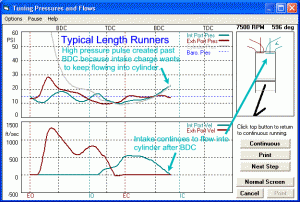
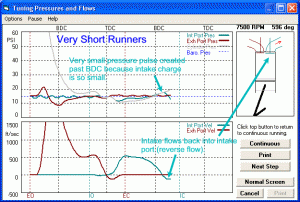
These tuning pulses are present in every running engine, because all engines have ports and runners to some degree. The size and duration of the exhaust suction pulse during TDC overlap, and the intake pressure pulse at intake closing depend on many things, like length and diameter of the runners and header primary, cam timing, piston speed, etc. That is why some engines will only get to 65% volumetric efficiency at full power and others will get to 130%. However, it should now be easy to see how you can actually be better than perfect.
Note: There is more to intake and exhaust tuning pulses than just the inertia effect. However, these other effects are beyond the scope of this blog. The good news is that the Engine Analyer Pro takes all these tuning effects into account when doing its calculations. Then all you have to do is check the torque and HP curves, because that is still the bottom line: how much torque and HP do all the tuning effects produce at all the various RPMs.